Neutrinos rarely interact, so for DUNE to catch lots of neutrinos, it will need to be fed an incredible number of these particles. LBNF will generate trillions of neutrinos every second using Fermilab’s powerful accelerators, improved by PIP-II.
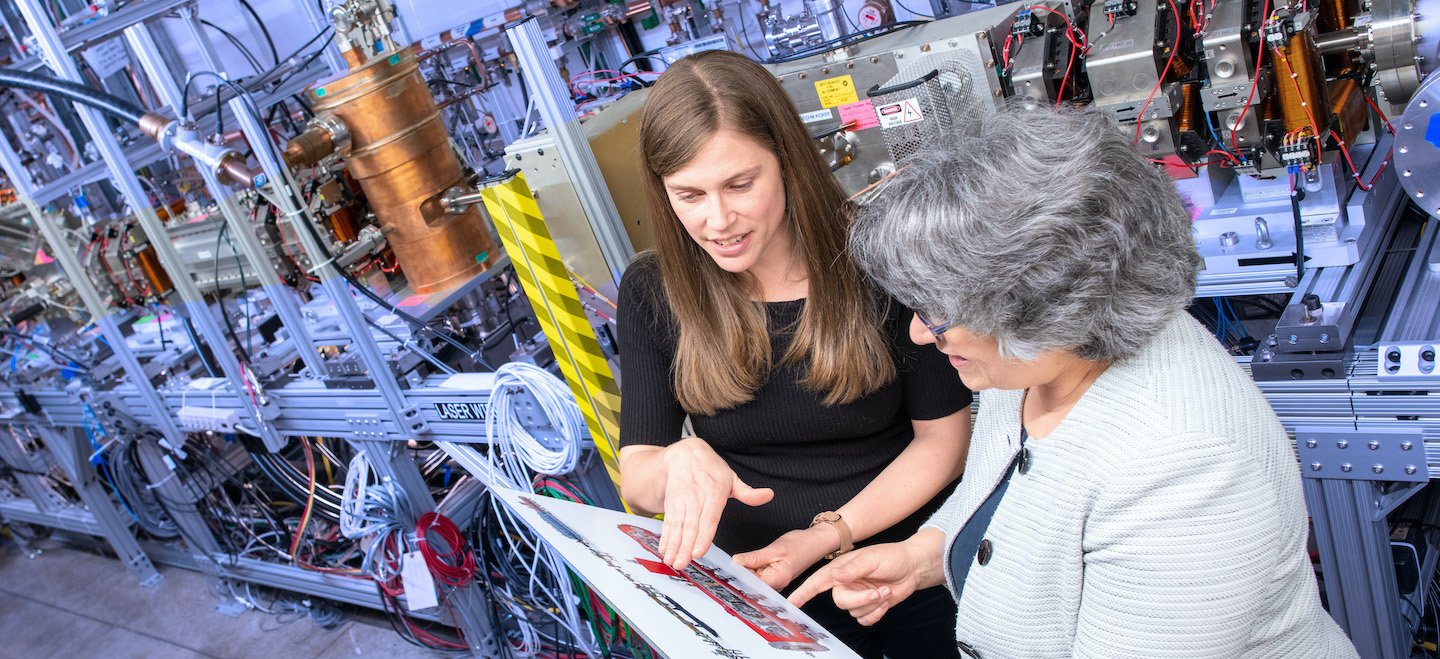
How to make a neutrino beam
This two-minute animation gives a quick overview of how the process works.
So how does LBNF make a neutrino beam?
The process will start in a new particle accelerator called PIP-II. Here, state-of-the-art superconducting cavities will efficiently accelerate protons to up to 800-million-electronvolts as they travel through the 215-meter-long (700-foot-long) machine.
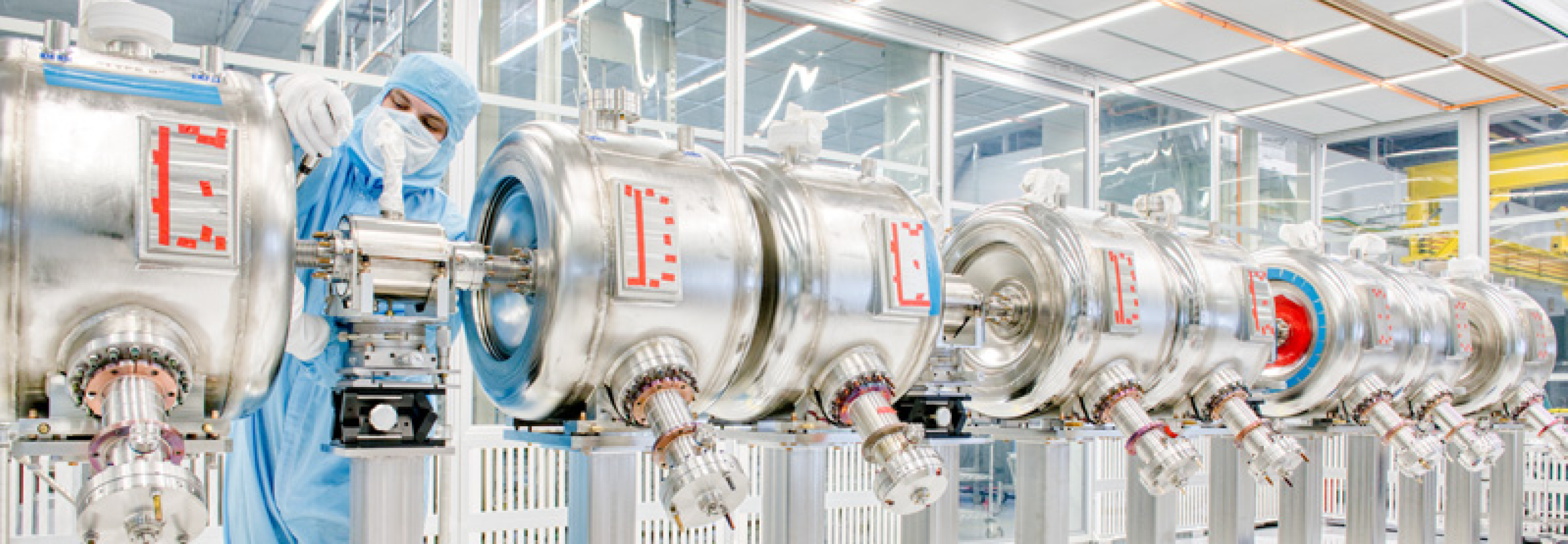
This powerful proton beam will gain more energy as it proceeds through Fermilab’s chain of particle accelerators, moving to the Booster for an extra kick, reaching up to 8-billion-electronvolts, and then on to Fermilab’s largest accelerator, the Main Injector, which will accelerate the protons to even higher energies: 120-billion-electronvolts. Once it exits the final accelerator in the chain, the beam of protons will move almost as fast as the speed of light and carry more than 1 megawatt of energy.
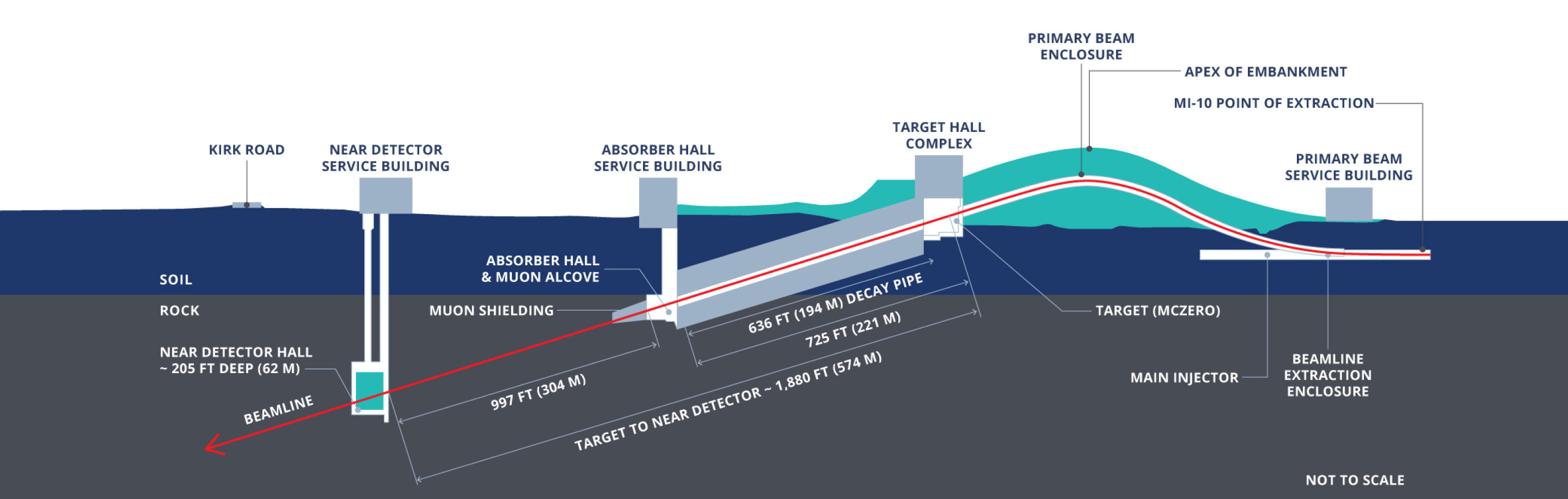
The protons will then be extracted from the Main Injector and precisely directed toward the DUNE detectors. Traveling through a brand new beamline, they will finally smash into a cylindrical rod of graphite called the target.
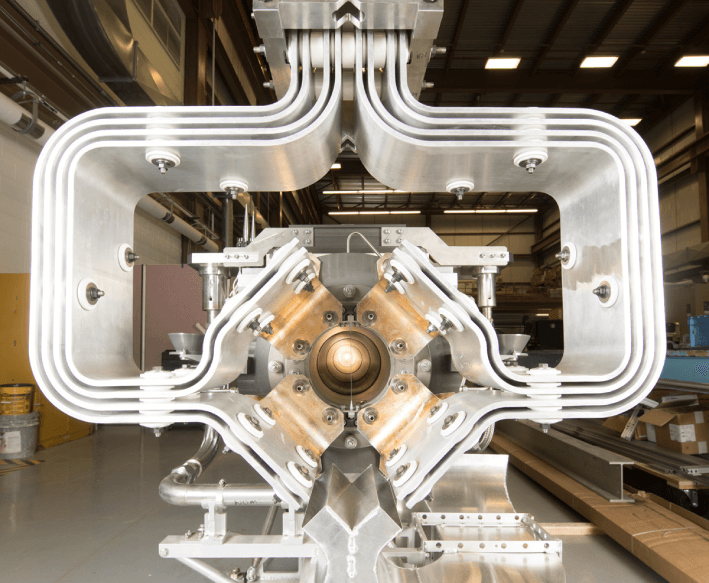
The protons’ collisions with the target produce other particles with electric charge that can be quickly focused into a tight beam by a series of magnets called horns. Those new particles decay into neutrinos that can no longer be directed by magnets, but that will keep traveling, ghostlike, through matter. The neutrinos pass through a detector on the Fermilab site, then through 1,300 kilometers (800 miles) of earth and then the enormous liquid-argon detector underground in South Dakota. The distance between the near and far detector, called the baseline, provides maximum sensitivity to the properties scientists want to measure.
By switching the horns to select and focus particles with the opposite electric charge, scientists can also produce a beam of the neutrinos’ antimatter counterparts, antineutrinos.
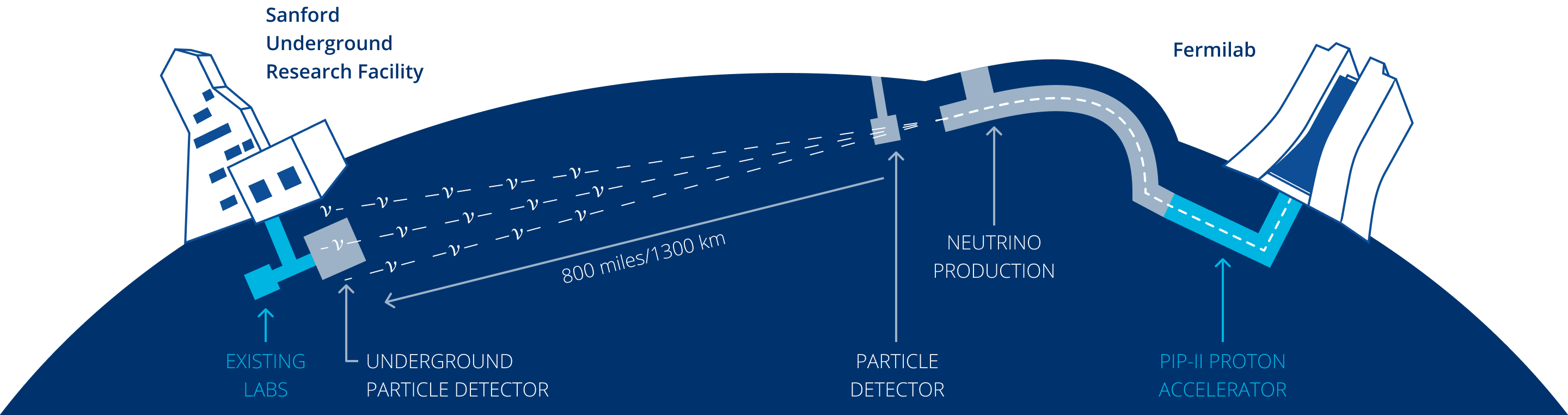
Neutrinos come in three types: electron, muon and tau. Initially, almost all of the neutrinos and antineutrinos in the beam that leaves Fermilab are of the muon variety. Then they change type — or oscillate — as they travel.
Like a beam of light from a flashlight, the neutrino beam will widen over distance. To make sure that the largest possible number of neutrinos arrives at the detector hundreds of miles away, scientists must make sure that the particle beam is concentrated, focused and aimed just right when making the neutrinos.